Kraken 15at сайт krakens15 at
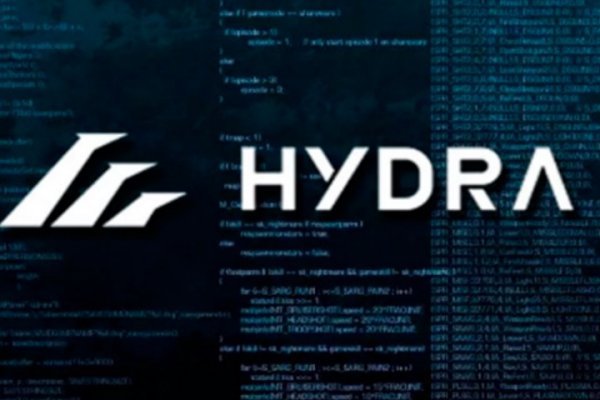
Onion-сайты v2 больше не будут доступны по старым адресам. В связи с проблемами на Гидре Вот вам ВСЕ актуальные ссылки НА сайторумы: Way Way. Годный сайтик для новичков, активность присутствует. Английский язык. Всяческие политико-революционно-партизанские ресурсы здесь не привожу намеренно. Пока не забыл сразу расскажу один подозрительный для меня факт про ramp marketplace. "При обыске у официальный задержанных обнаружено и изъято наркотическое средство мдма массой 5,5 тыс. В том меморандуме платформа объявила о выходе на ICO, где 49 official «Гидры» собирались реализовать как 1,47 миллиона токенов стартовой ценой 100 долларов каждый. Действует на основании федерального закона от года 187-ФЗ «О внесении изменений в отдельные законодательные акты Российской Федерации по вопросам защиты интеллектуальных прав в информационно-телекоммуникационных сетях». Ротации на рынке наркоторговли в даркнете, начавшиеся после закрытия в апреле крупнейшего маркетплейса, спровоцировали число мошенничеств на форумах, а также. Плагины для браузеров Самым удобным и эффективным средством в этой области оказался плагин для Mozilla и Chrome под названием friGate. Crdclub4wraumez4.onion - Club2crd старый кардерский форум, известный ранее как Crdclub. Onion - Verified зеркало кардинг-форума в торе, регистрация. Робот? Оригинальное название mega, ошибочно называют: mego, мего, меджа, union. Купить через Гидру. Потребитель не всегда находит товар по причине того что он пожалел своих денег и приобрел товар у малоизвестного, не проверенного продавца, либо же, что не редко встречается, попросту был не внимательным при поиске своего клада. Главный минус TunnelBear цена. С другой стороны, у него есть версии для iOS, Android, PC и Mac: последние две очень простые в использовании. Частично хакнута, поосторожней. Какой же функционал нам представляет Matanga? Onion - WeRiseUp социальная сеть от коллектива даркнет RiseUp, специализированная для работы общественных активистов; onion-зеркало. Первый это пополнение со счёта вашего мобильного устройства. Этот сайт упоминается в деловой социальной сети LinkedIn 0 раз. По мне же, так удобнее изменить путь и распаковать его в специально подготовленную для этого папку. Onion - Darknet Heroes League еще одна зарубежная торговая площадка, современный сайтик, отзывов не нашел, пробуйте сами. Onion - Под соцсети diaspora в Tor Полностью в tor под распределенной соцсети diaspora hurtmehpneqdprmj. Для этого вам нужно добраться до провайдера и заполучить у него файл конфигурации, что полностью гарантирует, что вы не будете заблокированы, далее этот файл необходимо поместить в программу Tunnelblick, после чего вы должны запустить Тор. Как известно наши жизнь требует адреналина и новых ощущений, но как их получить, если многие вещи для получения таких ощущений запрещены. Читайте также: Что делать если выключается ноутбук от перегрева. Правильное названия Рабочие ссылки на Мегу Главный сайт Перейти на mega Официальное зеркало Зеркало Мега Альтернативное зеркало Мега вход Площадка Мега Даркнет mega это каталог с продавцами, маркетплейс магазинов с товарами специфического назначения. Отойдя от темы форума, перейдем к схожей, но не менее важной теме, теме отзывов. Как зарегистрироваться на Mega? Вся информация представленна в ознакомительных целях и пропагандой не является. Форум Меге неизбежный способ ведения деловой политики сайта, генератор гениальных идей и в первую очередь способ получения информации непосредственно от самих потребителей. Торрент трекеры, библиотеки, архивы. Чаще всего они требуют всего лишь скопировать ссылку в строку на своей главной странице и сделать один клик. Что-то про аниме-картинки пок-пок-пок. Zcashph5mxqjjby2.onion - Zcash сайтик криптовалютки, как bitcoin, но со своими причудами. Вместо курьера вы получите адрес и описание места где забрать заказ. Почему именно mega darknet market? p/tor/192-sajty-seti-tor-poisk-v-darknet-sajty-tor2 *источники ссылок http doe6ypf2fcyznaq5.onion, / *просим сообщать о нерабочих ссылках внизу в комментариях! Onion - the Darkest Reaches of the Internet Ээээ. У каждого сайта всегда есть круг конкурентов, и чтобы расти над ними, исследуйте их и будьте на шаг впереди.
Kraken 15at сайт krakens15 at - Что такое даркнет кракен
Иногда создаётся такое впечатление, что в мировой сети можно найти абсолютно любую информацию, как будто вся наша жизнь находится в этом интернете. Частично хакнута, поосторожней. Еще один способ оплаты при помощи баланса смартфона. Mixermikevpntu2o.onion - MixerMoney bitcoin миксер.0, получите чистые монеты с бирж Китая, ЕС, США. Начинание анончика, пожелаем ему всяческой удачи. Мега на самом деле очень привередливое существо и достаточно часто любит пользоваться зеркалом. Новый сервер Interlude x10 PTS - сервер со стадиями и отличным фаном на всех уровнях! Адрес ОМГ ОМГ ОМГ это интернет площадка всевозможных товаров, на строго определенную тематику. Что такое брутфорс и какой он бывает. Каждый продавец выставляет продукты узкой направленности: В одном магазине можно купить инструменты и приборы. Форумы. Есть три способа обмена. Форум Форумы lwplxqzvmgu43uff. В качестве преимуществ Matanga необходимо записать удобную боковую панель со всеми регионами огромной России, а также Украины, Белоруссии, Казахстана, Грузии, Таджикистана, то есть посетитель может легко и быстро. Респект модераторам! Клёво2 Плохо Рейтинг.60 5 Голоса (ов) Рейтинг: 5 / 5 Пожалуйста, оценитеОценка 1Оценка 2Оценка 3Оценка 4Оценка. Ml -,.onion зеркало xmpp-сервиса, требует OTR. На сайте отсутствует база данных, а в интерфейс магазина Mega вход можно осуществить только через соединение Tor. Ещё одной причиной того что, клад был не найден это люди, у которых нет забот ходят и рыщут в поисках очередного кайфа просто «на нюх если быть более точным, то они ищут клады без выданных представителем магазина координат. Перемешает ваши биточки, что мать родная не узнает. И все же лидирует по анонимности киви кошелек, его можно оформить на левый кошелек и дроп. Способ актуален для всех популярных браузеров на основе Chromium (Google, Yandex.д. Хороший и надежный сервис, получи свой.onion имейл. Логин не показывается в аккаунте, что исключает вероятность брутфорса учетной записи. Различные тематики, в основном про дипвеб.
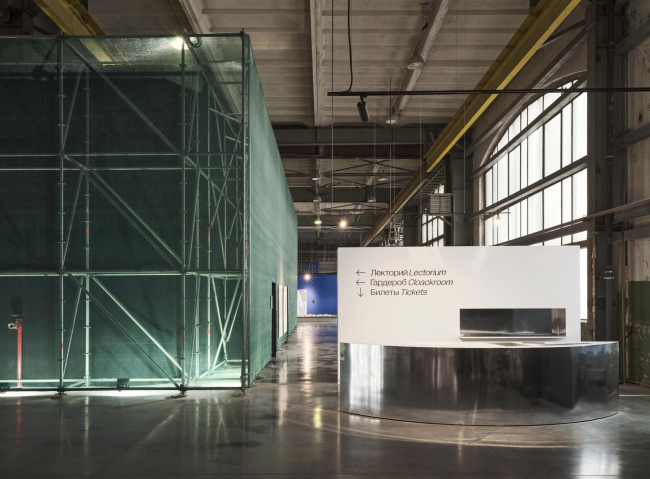
Мы победили. При покупке: если эта цена ниже последней рыночный цены, ваш лимитный ордер добавляется в стакан заявок. Даже если вы перестанете использовать Freenet. Причём это в той ситуации, что за два уже года жизни ребёнка со мной, мать практически ни разу не интересовалась жизнью дочери и не помогала материально. Суд проходил в колпинском районе СПб, Судья Суворова. 4.6/5 Ссылка TOR зеркало Ссылка https shkaf. Является зеркалом сайта fo в скрытой сети, проверен временем и bitcoin-сообществом. I2P должна быть скачана и установлена, после чего настройка осуществляется через консоль маршрутизатора. Администрация форума активно сотрудничает с властями сразу нескольких государств).4/5 Ссылка TOR зеркало Ссылка TOR зеркало http xssforumv3isucukbxhdhwz67hoa5e2voakcfkuieq4ch257vsburuid. Как я писал выше, важным неприятным моментом было то, что дочь действительно просела по успеваемости (к моменту процесса мы уже учились очно, в другой школе по месту жительства, но оценки по некоторым предметам шли к двойкам в четверти, выправлять не всегда успевали). Пытаться интересоваться начала только когда в суде на заседании у неё спросили, а вы вообще интересуетесь жизнью своего ребёнка? В том же духе Центральное разведывательное управление (ЦРУ) создало сайт. Становится доступной спотовая и маржинальная торговля. Нарния площадка ориентированная преимущественно на марихуану, так же легкие «partydrugs». Он назначает задачу клиенту, а не серверу, устраняя любую уязвимость, связанную с сервером. Onion CryptoParty еще один безопасный jabber сервер в торчике Борды/Чаны Борды/Чаны nullchan7msxi257.onion Нульчан Это блять Нульчан! Прямая ссылка: https thehidden. Цель сети анонимности и конфиденциальности, такой как Tor, не в том, чтобы заниматься обширным сбором данных. А более широкое распространение он получил в 2002 году, благодаря публикации работы программистов Microsoft, описывающей принципы работы даркнета. Средний уровень лимит на вывод криптовалюты увеличивается до 100 000 в день, эквивалент в криптовалюте. Для прохождения среднего уровня необходимо пройти базовый уровень и дополнительно предоставить: справку о вашем роде занятий, удостоверение личности, подтвердить адрес проживания, предоставить фото с документом и надписью Для Kraken текущая дата. На конкретизирующих вопросах от суда или адвоката всё прояснялось. Onion PIC2TOR, хостинг картинок.